Achieving the world’s ambitious climate goals will require rapidly scaling economically viable technologies, while also innovating, incentivizing, and investing in less mature technologies that are not yet cost-competitive.
1Special thanks to Ian Samuels at New System Ventures, Landon Derentz and Reed Blakemore at the Global Energy Center of the Atlantic Council, and Nishant Tiwary for their meaningful contributions to this report.
In the past, we examined how global emissions are distributed across companies and sectors and the role that investors and governments play in decarbonization. In this report, we turn our attention to the specific climate technologies that are critical for achieving net zero goals and where they are in terms of their technological and commercial maturity. This matters for investors; 75% of publicly traded companies globally (and 60% of all high-emitting companies) have released low-carbon transition plans. A central question regarding the transition to a low-carbon economy is: will high-emitting companies and sectors have the necessary technology to transition, at acceptable cost?1
We estimate that about 40-50% of global emissions reductions required to achieve net zero goals can come from scaling technologies that are already mature. These technologies are proven and cost-competitive enough to be deployed at commercial scale (e.g., solar, wind, electric vehicles, heat pumps). Some subsidies or incentives are still likely required to get the switch to happen in a timely way and at scale. This is because the extensive build-out of low-carbon technologies and replacement of high-carbon legacy physical assets, even if economically viable, is not necessarily so profitable that companies will want to do it immediately and without policy inducement. It will depend on typical capex considerations—return on investment relative to the cost, age, and turnover of existing assets, project execution, economies of scale, availability of materials, policy backdrop, capital access, etc. Climate policy support through measures such as the Inflation Reduction Act in the US, the Net Zero Industry Act in Europe, and support for strategic sectors in China have greatly enhanced the pace of innovation and investment in adopting these technologies at scale. That said, the scaling up of these technologies is going too slowly to meet the world’s ambitious climate goals. We estimate there are large financing gaps. Roughly $3-5 trillion a year (around 4% of global GDP) is needed to scale these technologies against the roughly $1.5 trillion a year that is currently being deployed. The financing shortfall is largest in emerging markets.
The technology is not yet there to address the remaining 50-60% of emissions, though innovation is happening rapidly. To address the remaining emissions, solutions such as green hydrogen, direct air capture, sustainable aviation fuel, and nuclear fusion (or others yet to be invented) will be necessary, but significant technological progress is required before they become commercially feasible and economically viable. Here it is harder to estimate how much capital will be necessary to successfully operationalize these technologies, but it is unlikely that the roughly $500 billion a year currently being invested to develop these earlier-stage technologies is sufficient to make the necessary progress (especially since many are at the prototype phase, and more capital will be needed once the technologies are ready to scale). Unproven technologies are risky, and thus require more risk-tolerant capital (e.g., venture capital, project finance, philanthropic support) and project de-risking (e.g., government support, blended finance) alongside in-house capex efforts of large corporate players.
High-emitting sectors make up 30% of global public equity market capitalization and represent critical components of global and national economies. So, the transition to a low-carbon economy will have large impacts, including on corporate capex and global flows of capital, supply and demand patterns for key inputs, and national and industrial competitiveness. The table below shows the maturity of technologies needed to reduce or eliminate emissions in these sectors and emissions categories. By “mature,” we mean technologies that are proven and past the demonstration stage, and where technological improvements have reduced costs to a point where low-carbon technology can be realistically scaled up without a prohibitive cost differential to existing higher-carbon technologies.

In the first half of this report, we discuss the landscape of climate technology and how the world is tracking against its emissions reduction goals. In the second half, we then share supplementary assessments of the state of key decarbonization technologies.
To level set, the transition to a low carbon economy is not on track to meet the world’s ambitious goals. CO2 emissions need to fall around 50% by 2030 and to net zero by 2050 in order to contain the global temperature increase to 1.5°C. While emissions have stabilized, they have yet to start falling. And while we acknowledge that there is a very wide range in estimates, by almost any measure the actual amount of money going into developing and deploying technologies that will reduce emissions is far from what is needed to enable such a steep reduction.

That said, significant progress has been made over the last decade as low-carbon technologies have matured and begun to be rolled out rapidly. Rapid innovation has led to decreases in the cost of technologies like solar, wind, and electric vehicles, all of which are now competitive with fossil-fuel-based products. As with innovation in other technologies, experimentation and real-world application, economies of scale, greater technical know-how, and improvements in production improve the efficiency and economics. For example, solar modules have undergone around a 20% “learning rate” over the past two decades (IRENA data), falling in cost by 20% each time capacity is doubled.
Much of the flow of capital so far has gone into scaling these mature technologies, as the investment case is stronger when comparing mature green tech against high-carbon incumbent alternatives. For example, innovation pushing down the cost of wind turbines has increased the internal rate of return of wind farms to be similar to that of coal plants. Because of their growing profitability, investment in renewables now surpasses investment in fossil fuels by a factor of 1.8, according to IEA data, with solar and wind capacity scaling faster in energy generation terms than natural gas or nuclear, when they first came online.
Though the low-carbon transition is motivated by environmental—and increasingly energy security—considerations, it is policy induced. Unlike other technological transformations, the low-carbon transition needs to be rolled out at an accelerated pace to meet global climate goals. Many carbon-intensive capital assets have low turnover rates. So even if the new technologies are mature and economically viable, they are not necessarily so profitable that consumers or companies will shift immediately, and many players will need policy inducement (either in “carrot” or “stick” form) to take steps consistent with the necessary pace of transition. For example, even if EVs are cost-competitive with internal combustion engine cars, households that still own working or relatively new ICEs aren’t likely to buy a new EV immediately—a subsidy or incentive likely is still a required impetus to get the switch and upfront spend to happen in a timely way.
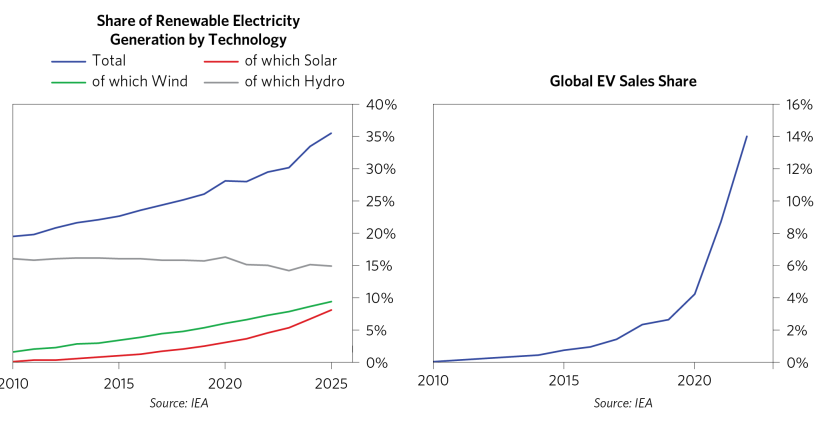
As these relatively mature climate technologies roll out, there are significant economic impacts: the creation of new large publicly traded companies (e.g., Tesla, First Solar), changes to greener business models in existing companies (e.g., Mercedes-Benz rolling out EVs from ICEs, Orsted shifting from primarily coal to primarily wind generation), rising demand for key inputs (e.g., commodities necessary for green tech such as copper, nickel, lithium, cobalt), and altered geopolitical dynamics (e.g., Chinese dominance in solar panel manufacturing and its implications).
By contrast, less mature technologies need earlier stage investments in order to meet the promise of meaningful emissions reductions at scale. As shown below, even risk-tolerant venture capital’s breakdown of climate investment has been much more heavily concentrated in mature technologies such as transport, energy, and buildings (around 70% of funding since 2020). By contrast, sectors like food and land use, industry, and carbon capture, where technologies are less mature, remain underfinanced relative to their emissions-reductions potential. To get sufficient investment in sectors where the technological road map is less clear will likely require more subsidies, incentives, and/or philanthropic capital.

Countries are racing for leadership in climate technology via supportive green tech policies. From a geopolitical standpoint, climate technology (like AI) represents critical infrastructure for the 21st century. Accordingly, policy makers are reluctant for others to have dominance in the technology, and leadership in these areas will likely serve as an engine of strategic economic growth. From a transition standpoint, these competing supportive policy regimes are facilitating the development and rollout of low-carbon technologies.
- China: Climate tech has been a strategic priority for China for decades, with policy makers providing generous support since the mid-2000s (e.g., production subsidies). This has succeeded in galvanizing innovation in the “new three” of solar cells, lithium batteries, and electric vehicles. Across the “new three,” Chinese companies today control a huge share of global production capacity and have been able to maintain this high market share by substantially outcompeting products from other countries based on the low costs and high quality of their manufacturing. For example, China accounts for around 80% of the world’s solar manufacturing capacity, while alternatives in other countries are fewer and much more expensive (US panels remain almost 40% more expensive even after US subsidies and tariffs). China also leads the world in producing EVs and in the EV parts, batteries, and minerals supply chain. BYD, the largest Chinese EV manufacturer, produced 3 million battery and hybrid EVs in 2023, much higher than Tesla’s 1.8 million. Looking ahead, China’s Five-Year Plans continue to include low-carbon development and technological innovation and advanced manufacturing as core tenets of their strategy, with policy frameworks such as the “30/60” and “N+1” enabling decarbonization across a range of energy and industrial sectors. China’s competitive edge has galvanized other countries to action, with some increasing support for domestic green production while others step up anti-China protectionist policies to defend their own green supply chains.
- USA: The Inflation Reduction Act (IRA) is a transformative climate bill that has provided strong support for the US clean-tech industry across both mature and emerging climate technologies. Overall, the US has seen a boom in green tech investment since the IRA’s announcement. In solar, it has already galvanized over $100 billion of investment and created approximately 20,000 manufacturing jobs, while clean-electricity tax credits have also benefited consumers, utilities, and states. The ripple effects of the IRA are also evident in the energy storage domain, where companies are now charting growth trajectories that extend beyond traditional equity financing. While challenges remain around implementation and permitting, as well as the exact criteria for companies to qualify for certain subsidies, the US continues to attract new capital investment across both mature technologies—with companies like Linde, First Solar, Ford, and Enphase adding domestic manufacturing capacity in sectors such as renewables and electric vehicles—and more early-stage projects—such as investments in clean-hydrogen facilities by Linde and Air Products and the construction of the world’s largest STRATOS direct-air capture plant. However, politics will play an important role in the longer-term trajectory of US climate policy, and how supportive it remains to new technologies.
- Europe: Europe’s climate strategy is shifting gradually from a “stick” to “carrot” approach; having historically focused on carbon pricing via the EU ETS (Emissions Trading Scheme), it has recently ramped up subsidy-based programs, partly as a response to similar green fiscal measures in the US (especially the IRA). Europe’s mix of policies has succeeded in scaling up deployment of mature green tech (renewables, EVs) and positioned it as an early tech leader in decarbonizing the industrial sectors. For example, REPowerEU (launched in the wake of the Russia-Ukraine conflict) increased investment in renewables as a means of increasing energy independence; Fit for 55 included consumer-directed subsidies for EVs; and the Net Zero Industry Act is expected to help unify and streamline country-level “carrot”-type policies that diverge in terms of their subsidies to green technology, regulation, and permitting, as well as setting a 40% manufacturing target of strategic net zero technologies. With structural changes in access to natural gas, this renewable policy path will be critical for determining the competitiveness of their industrial base going forward. In less mature technologies, Europe is handing out huge green innovation grants to CCUS and industrial projects (e.g., Heidelberg’s GeZero green cement project or ExxonMobil’s carbonate fuel cell CCUS plant). As a result, European companies lead the world in researching lower-cost and scalable CCUS technologies, while the Biden administration recently awarded government investments to a handful of European industrials to help build out the US’s low-carbon steel, cement, and chemicals sectors (SSAB, Heidelberg, Orsted).
Many climate technologies tend to be capital-intensive, with high upfront costs and longer project durations. Building a renewable plant requires the expensive purchase and installation of equipment such as turbines and solar panels, and development can take multiple years before the plant begins generating revenues. Wind power, for example, has higher capex (but lower ongoing operating expenses) compared to operating legacy high-carbon infrastructure. For emerging technologies, this dynamic is exacerbated as future payouts are more uncertain (e.g., how much of a green premium will customers be willing to pay for low-carbon steel or cement and will end uses of hydrogen expand to new sectors such as transportation or utilities). As such, climate-tech producers and implementers often have larger upfront capital requirements and have a larger share of their earnings discounted into the future. These high upfront costs also limit the pace of adoption of green tech, even for mature technologies that are cost-competitive over their entire lifespan.

As such, in a rising interest rate environment, as we have seen in the past year, clean-tech projects are hit harder by high borrowing and debt financing burdens. As a result of capital intensity and long duration, their rate of return, future earnings, and valuations are hit harder by increasing discount rates. For example, Danish wind power leader Orsted was forced to cancel a $2.4 billion US wind plant project as rising rates and projected debt-financing costs rendered the project unprofitable, which was compounded by shortages and inflation in the offshore wind supply chain. This led to huge balance sheet impairments and a collapse in stock pricing of more than 50% peak-to-trough in 2023. Likewise, capital-intensive offshore wind investment plunged when European rates rose in 2022, and climate tech VC funding has fallen since the post-COVID high-rate regime.
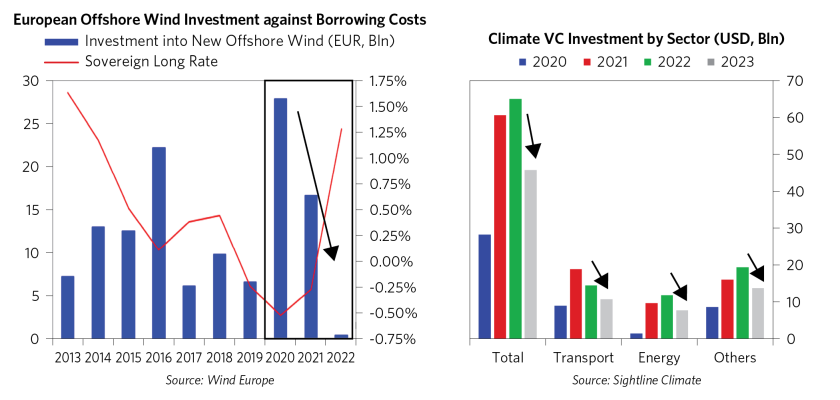
The impact of high interest rates is especially pertinent in emerging markets, which already face the biggest financing shortfall. On average, emerging market countries face higher costs of capital for green tech investments than developed markets (around 10% versus 5%). According to the IEA, financing costs for renewable energy projects in emerging markets can make up more than 50% of lifetime costs, compared to 30-40% in Europe or the United States. These high costs compound the risks already inherent in climate technologies—even those on the higher end of the maturity spectrum—and on balance create larger hurdles to investment for climate tech in emerging markets. In addition to cost, the volume of capital in emerging markets has not been adequate to support decarbonization: most recently, the COP28 global stocktake highlighted the shortfall in global efforts to fund climate mitigation and adaptation in emerging markets, including relative to prior commitments.
It is key for investors to keep track of the rapidly evolving “opportunity set” of climate technologies, which will over time enter the public market realm as they reach cost-competitiveness and scale up. As we described in a previous report 90% of corporate emissions and 60% of global emissions come from just 11 emissions-intensive sectors. In each of these high-emitting sectors, there is a wide spectrum of technologies ranging from mature and commercially scalable alternatives (e.g., solar, wind, electric vehicles) to emerging technologies that are not scalable yet but that are likely to grow rapidly in the coming years (e.g., sustainable aviation fuels, green hydrogen, direct air capture) with the support of government policies, continued innovation, and risk-tolerant capital. Of course, technologies are not monolithic and there are specific considerations for every project, including national policy, surrounding infrastructure, domestic cost of capital, etc., leading to large differences in the deployment of technologies across countries.
The chart below shows the relative emissions-reduction potentials of these technologies, broken down into five distinct categories that we think help to synthesize the options available:
- Switching to renewable energy from fossil fuels
- Electrifying processes that rely on decentralized fuels
- Improving resource utilization, efficiency, and recycling
- Re-engineering carbon-intensive industrial processes through new technologies or chemistry
- Promoting carbon capture, utilization, and storage, including other GHGs such as methane
In other words, emissions reduction can be conceptualized as requiring a combination of (1) stopping the use of fossil fuel energy generation (e.g., go to solar), (2) electrifying processes with the new low-carbon energy (e.g., switch to electric vehicles from internal combustion engines), (3) cutting the emissions intensity of resources used (e.g., recycling), (4) finding new ways to decarbonize remaining sources (e.g., sustainable aviation fuel), and (5) capturing the remaining emissions (e.g., carbon capture).
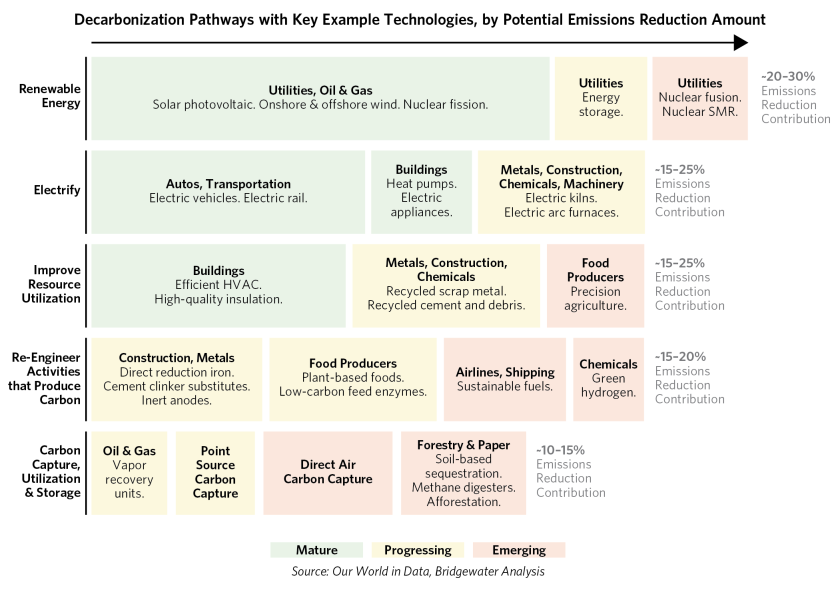
A successful climate transition will require concerted action across all these avenues: some of these (e.g., renewable energy, electrification, and resource utilization) tend to be more mature and support emissions reductions in public companies in sectors such as utilities, buildings, and autos; while others (e.g., re-engineering processes and capturing carbon) are more complex technically and not yet scalable, but will be needed for emissions reductions in hard-to-abate sectors like metals, cement, chemicals, aviation, and shipping.
As a methodological note, our analysis is designed to give an indicative top-down sense of the distribution of potential emissions reduction across sectors and technological readiness. They are based on our research on each key decarbonization technology across all high-emitting industries. Because it is difficult to quantify precisely how much specific technologies can cut emissions, we have aggregated up into the five broad avenues described above. There is imprecision inherent in this analytical exercise: ambiguities exist in the classification of mature versus non-mature technologies (we consider “mature” those technologies that are proven past demonstration phase, and have reduced costs to a point where they can be realistically scaled up) as well as in pathways that chart how much specific technologies can reduce future emissions. And some of these technologies will likely compete with each other (e.g., electrification versus hydrogen in industry), so (among other reasons) there is no precision in sizing emission reductions or capital needed.
In the remainder of this report, we go through a supplemental two-page overview for each of the five decarbonization pathways with summary discussions of each component in the above diagram. This section is intended for readers interested in overview assessments of the key climate technologies. We acknowledge that each of these technologies is in itself an entire discipline with considerable nuance and therefore our treatment of each is brief and introductory.
I. Renewable Energy: A Terawatt Opportunity with Room for Growth in Storage and Nuclear Fusion

Technological innovations have driven huge reductions in renewable energy costs, and many renewables are today competitive with, or even cheaper than, fossil fuels on a levelized cost of energy (LCOE) basis. Renewables are receiving huge financing flows of over $500 billion annually and accounted for around 85% of added global power capacity in 2023. Most of this capital is coming from traditional capex channels such as debt, equity, and bank lending, and many of the projects are undertaken by existing incumbent power producers. Renewables are now around 12% of the global energy mix, but getting the global energy mix to the IEA target of around 30% renewable share by 2030 will still require a tripling of global renewable capacity. Relative to decarbonization goals, the largest investments are needed in countries with coal-reliant grids like China and India. Even in mature technologies, streamlined permitting processes, upgraded transmission infrastructure, and grid-scale battery storage are needed to unlock further investment. Streamlining the energy permitting process is especially crucial—more than two terawatts of clean energy capacity are currently awaiting integration into the US transmission system, surpassing the amount of clean energy already installed.


Solar photovoltaic technology is cost-competitive and scalable. Its main barrier is no longer technological, but the availability of grid-scale energy storage. Thanks to rapid innovation over the last decades, especially in China, solar LCOEs have fallen 90% since the 2000s and are now cheaper than fossil fuels on an LCOE basis. Solar LCOEs are likely to fall slightly further in the next decade, with continued innovation in cheaper tracking systems, perovskite solar cells, and increased automation and digitalization in the operation of solar farms. This has contributed to a doubling of installed solar capacity in the last three years (over 1.5 terawatts), with China making up 50% of this increase. However, energy storage needs to increase in pace with solar capacity. Because solar is available only in the daytime, cheap and efficient batteries are needed to level the timing mismatch between solar availability and electricity demand and are crucial in ensuring the profitability and stability of solar project earnings.
Strong innovation has reduced the costs of both onshore and offshore wind by about 60% since the 2010s, although onshore wind technology is cheaper and more mature than offshore wind technology today. In general, offshore wind tends to be more efficient due to the higher speeds and greater consistency of winds, but requires more complicated infrastructure and maintenance, leading to higher upfront and operating costs. There are also large geographic differences, with offshore wind being more competitive in Northern Europe. However, as described earlier, both are hitting supply chain constraints and borrowing-cost problems due to higher interest rates affecting their internal rate of return.
A viable scale-up of solar and wind must be paired with grid-scale energy storage, but storage technologies (e.g., batteries) are currently less mature and require stronger policy incentives. While the operating costs of wind/solar are relatively low, returns on renewables projects are hurt by their tendency to cluster together and compete with each other in the same spaces (where land is cheap) and times (during sunlight or windy conditions). This also makes them less effective as an energy “baseload” that provides consistent and dispatchable electricity throughout the day. Grid-scale storage and expanded transmission grids ease the geographic and temporal concentration of renewable energy, by allowing energy to be transported or stored for future use. However, despite lithium battery prices falling 90% since 2010, more innovation is required to cut the costs and increase the efficiency of storage technologies, especially in long-term storage with capacities of over four hours.
Geothermal energy is mature and cheap, but its widespread use is limited by the natural availability of geological heat reservoirs, its high upfront costs, and barriers to land access. Geothermal plants extract heat from the earth and convert it into electricity, with LCOEs similar to solar and wind. The most common types, flash steam and dry steam plants, extract steam or hot water from underground reservoirs and pipe it to turbines that generate electricity. Though geothermal is a minimal (0.17%) share of today’s energy supply, the IEA estimates that it could account for about 3.5% of global electricity by 2050. In contrast to solar and wind, geothermal is dispatchable on demand, making it a promising source of renewable “baseload” electricity without the need to build out expensive battery storage. However, widespread use of geothermal energy is limited by the natural geological availability of heat reservoirs, which are usually found only near tectonic plate boundaries. In addition, drilling geothermal wells has extremely high upfront capital costs; its capital costs are higher than wind and solar plants, though its operating costs are lower. Today, geothermal is a sizable share of electricity production in only a handful of countries (e.g., Iceland, El Salvador, the Philippines), where heat reservoirs naturally exist in accessible locations.
Nuclear fission is increasingly recognized as an important decarbonization lever but must surmount political challenges and perceptions around public safety that limit its widespread adoption. Nuclear energy provides around 10% of the world’s power through 430 reactors today. This is set to expand, with 60 more plants under construction and 20 nations at COP28 committing to tripling capacity by 2050. China’s $440 billion nuclear buildout plan is especially ambitious, aiming to build out more than 150 new reactors in the next 15 years. Nuclear is an important existing power source in geographies such as France and Central/Eastern Europe (Slovakia, Ukraine, Hungary), where it makes up more than 50% of grid capacity. However, further expansion of nuclear is held back by high upfront capital costs and political challenges linked to public safety perceptions, as highlighted during recent discussions on nuclear’s eligibility under the EU Taxonomy. These obstacles could ease with recent innovations in small modular reactors (SMR). SMRs promise lower upfront costs, greater efficiency, and increased safety margins, but have yet to be deployed at full commercial scale.
Recent breakthroughs in nuclear fusion show its promise as a low-waste and low-cost energy source, but it is too early to tell if it will become a scalable and cost-effective climate technology. Fusion produces a huge amount of energy per unit mass (four times nuclear fission), and, unlike fission, does not produce long-lived radioactive waste. The National Ignition Facility (NIF) achieved breakeven controlled fusion ignition in December 2022 and has renewed optimism for developing a practical fusion energy system capable of generating net energy. A vibrant burst of startups (around 50, collectively raising over $6 billion) are exploring various techniques with the potential to overcome technical problems in managing extreme temperatures and the scarcity of tritium, which are key questions for commercial viability at scale.
II. Electrification: Mostly Scalable and Well-Funded, with Gaps in Industry

For a low-carbon global economy, products that rely on decentralized fossil fuels (e.g., combustion engine vehicles, gas boilers) must be re-designed to run on electricity and connected to the power grid. Because many of the technologies needed here are already mature and commercially scalable, electrification enjoys a relatively smaller financing gap, particularly in sectors like transport where many public companies are investing heavily in the space, supported by a mixture of “carrot” (e.g., production and consumer subsidies) and “stick” (e.g., ICE phaseout targets) policies. However, there is need for substantial development in heavy industry, where the technology is less mature today and business processes are very complicated. The precise scale of electrification also depends on the relative competitiveness of other green levers and technologies—for example, industrials can achieve emissions reductions via a mix of electrified furnaces, recycling, re-engineered industrial processes, and carbon capture, with the ultimate balance between these levers depending on their relative costs and feasibilities.


In industrial processes, electrification is progressing, but more innovation is needed in key technologies such as electric furnaces and kilns. Heavy industry (e.g., metals, chemicals, engines, machinery) is substantially complicated, expensive, and challenging to electrify. For example, steel production requires (traditionally coal-fired blast) furnaces to achieve temperatures above 1,000°C, a feat of engineering whose green alternatives (electric arc furnaces) are today more costly, limited, and nascent. While the vast majority of heavy industrial activity remains carbon intensive, major industrial public companies across the aluminum, steel, and chemicals sectors have taken strong steps to electrify production. For example, Tokyo Steel runs the world’s largest electric furnace, producing steel using recycled scrap steel. The electrification of industry as a decarbonization lever also depends on its relative competitiveness with other green levers. Industrial companies looking to go green today draw across a mixture of electric furnaces, recycled scrap or clinker inputs, or carbon capture, depending on their relative costs and feasibility.
In transport, electric vehicles are nearing ICE-cost competitiveness, with huge investments and innovations in charging infrastructure, resilience, and performance set to accelerate adoption rates. As discussed above, electric vehicles now represent about 15% of total auto sales. Many governments have set ICE phaseout targets, such as the EU’s 2035 target, and are supporting the transition via subsidies for the production and consumption of EVs. Thanks to a history of strong production subsidies that have taken the Chinese EV manufacturing industry to economies of scale, China today leads the world in cost-competitive EV production, with Chinese EVs making up almost 60% of global EV sales in 2023. Many large global auto producers across China, Europe, and the United States are also setting ambitious EV targets and allocating sizable amounts of their capex toward scaling up EV production. For example, BMW’s long-term strategy hinges on its Neue Klasse line, a series of next-gen EVs set to debut in 2025, while Rivian, Daimler, and Tesla are innovating in heavy-duty transport and trucks where fewer electric alternatives are available today. While EV supply looks to remain strong in the midterm, unlocking the EV demand to match requires a stronger policy push on building out charging infrastructure, cheaper EV production methods, and tech improvements on BEV range and resilience. At current rates of innovation, EV costs could fall about 40% over the next decade to become similar to, or even lower than, ICE costs. For example, Stellantis is investing across three battery startups to produce cheaper and more energy-dense EV batteries: Tiamat in sodium-ion batteries, Lyten in lithium-sulfur batteries, and Factorial in solid-state batteries. Charging infrastructure is a big part of the EV story. Particularly in the US and EUR, permitting to put chargers in is a major blocker to the scale-up of EVs. Outside of passenger vehicles, electrification is much harder for long-haul trucking, with exploration into technologies like battery swapping, hydrogen, and sustainable fuels.
In buildings, heat pumps are a profitable and low-risk investment for property owners to cut emissions and costs at the same time. Rather than generate “new” heat, heat pumps transfer heat from a source (e.g., surrounding air, water bodies, geothermal energy, waste heat from factories) to where it’s needed, such as indoor heating or boiling water. While heat pumps require upfront capital to install, they are much more efficient than combustion boilers and cut a building’s energy and operating costs, with a generally less than 10-year payback period. Policy has made heat pumps even more profitable by subsidizing installation costs, such as the IRA’s heat-pump specific tax credits and EU subsidies on household heat pump installations. Right now, Asia leads the world in heat pump adoption. In Japan, where the residential and industrial use of heat pumps have enjoyed strong historical subsidy support, 90% of homes are already equipped with heat pumps, as compared to 16% in the US. In the US, the REIT sector is investing increasingly in heat pumps. For example, SL Green, an office REIT, is installing heat pumps in its 11 Madison Ave office complex and expects a large operating cost reduction and around 10% lower energy costs for tenants.
III. Improving Resource Utilization: A Mixture of Mature and Early-Stage Technologies

Cutting the quantity of resources and production needed per unit of economic output can cut carbon emissions and costs at the same time. This can be achieved through measures such as recycling, which is especially pertinent given the high emissions intensity of extraction and limited resources available for commodities such as metals, which are needed for a wide range of climate technologies. In other sectors like buildings or agriculture, there are also efficiency gains that can be extracted from new technological developments such as efficient utilization or precision agriculture. This pathway has a mixture of mature and early-stage technologies (e.g., energy-efficient buildings can be a profitable business investment, while recycled cement is close in price to, but still not fully competitive with, brown cement).
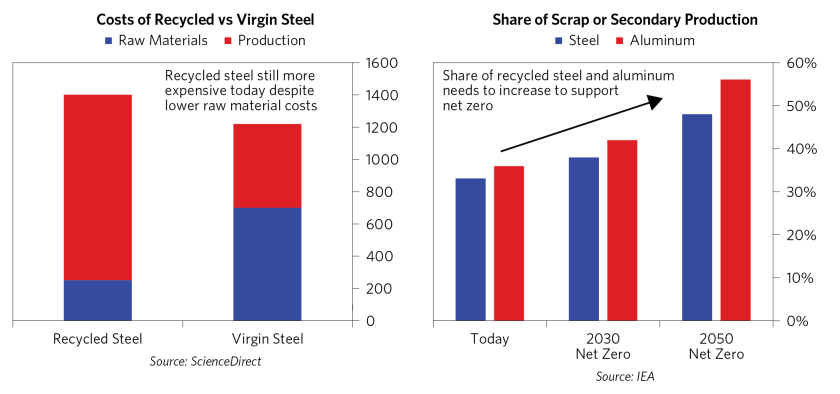
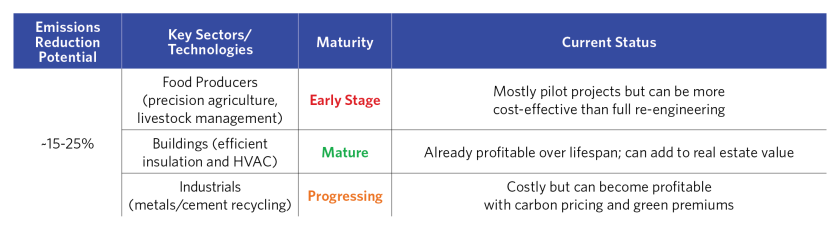
Agricultural emissions are difficult to abate with current technologies. Agriculture accounts for more than around 20% of total GHG emissions and a notable 40% of all human-caused methane emissions. The challenges are considerable: greenhouse gases are directly produced by hard-to-abate sources (e.g., livestock, traditional fertilizers, and land use). In addition, most of the world’s farming operations are small-scale and decentralized, meaning that changes in farming practices can be slow to implement at scale while their higher costs are borne directly by farmers who typically find it harder to pass costs on to corporate buyers with more market power. Agricultural climate technology is early-stage and underfinanced compared to other sectors, though there are promising developments in improving the carbon efficiency of agriculture using natural carbon sinks on farms and reducing food waste. For example, the government of India, as one of the world’s largest agricultural exporters, is building out an “Agristack,” a standardized database of agricultural data aiming to aid the digitalization of its agricultural sector, while the Agricultural Accelerator Fund it established in 2023 could potentially seed promising startups that accelerate the uptake of digital and precision agriculture.
Developing or refurbishing buildings to increase their energy efficiency can help to cut both emissions and costs, with technologies mostly mature and buoyed by a recent strengthening of policy support. Many of these technological investments (e.g., efficient insulation, HVAC) are already profitable; despite higher upfront costs, they generate energy-cost savings over time (by about 15%) with relatively short payback periods of less than a decade. Additionally, some REITs report that energy-efficient buildings now rent and sell for substantially (around 10-15%) more. Property owners are being spurred to action by strengthening energy codes in many countries, paired with subsidies for energy-efficiency renovations. For example, the US IRA offers households multiple tax credits for energy efficient home improvements, including up to an annual $1,200 for improving the insulation of walls, doors, and windows and installing more efficient air-conditioning and HVAC systems.
Using recycled materials in industrial processes is a costly but advancing lever for decarbonizing high-emitting industries such as metals, cement, and plastics. This can be achieved through measures such as recycling, which cut down emissions by optimizing material use and reducing the need for new extraction or production, which in sectors like cement or mining have large emissions impacts. The IEA estimates that the share of recycled steel and aluminum will need to increase to more than 50% of overall production (from about 35% today). Recycling processes are also gaining in importance as the amount of electronic or battery waste increases. Already, many large public companies are making investments in their recycling capabilities: Rio Tinto announced a $700 million investment in aluminum recycler Matalco, while Glencore partnered with Li-Cycle Holdings to use recycled materials for lithium-ion batteries. Cement is another industry where recycling can play an important role; while recycled cement currently costs about 25% more to produce than traditional alternatives—and more innovation is needed to reduce costs in the recycling, extraction, and production processes—the earnings of recycled cement makers can be supported by the green premium that some businesses have been willing to pay on green cement lines or by carbon pricing in certain geographies. Companies like Holcim (through their ECOPact cement line) are betting on recycling as a way to lower both their emissions and costs in the long term, reporting strong green demand and expecting the cost gap between its green and traditional cements to close with continued innovation and higher carbon pricing.
IV. Re-Engineering Zero-Carbon Alternatives: Early Stage, but Important in Hard-to-Abate Sectors

Some economic processes today directly release carbon into the atmosphere unrelated to the combustion of fossil fuels. For the world to reach net zero, such industries need to fundamentally re-engineer high-emitting manufacturing or chemical processes with more sustainable alternatives. This avenue currently needs much more front-loaded research and funding for mature technologies to be ready in time. Green alternatives are technologically difficult and early-stage, with riskier and longer-term payouts, leading to the large financing gap today. Most projects today are not cost-competitive without subsidies (e.g., green hydrogen), which creates challenges for the massive scale-up needed for net zero (e.g., building five times today’s hydrogen capacity and transitioning from fossil fuels as a feedstock to green alternatives such as water electrolysis).


Hydrogen has substantial potential as a green energy carrier. It can be produced with zero emissions via electrolysis, but scaling this up faces high-cost and infrastructural challenges. Because hydrogen can produce energy with zero emissions (either through combustion—which produces no carbon but can generate other air pollutants in the process—or in fuel cells), it could be used as a dispatchable clean-energy carrier if it is produced with renewable energy, though there is debate over how much it might be scaled. Most hydrogen today is produced using fossil fuels as a feedstock in the steam-methane reforming process (“gray hydrogen”), with only around 5% produced via carbon-free electrolysis (“green hydrogen”). A surge in supportive policy in the US and Europe has led to an uptick in the green hydrogen pipeline. For example, Air Products has committed about 20% of its historical PPE toward capex for low-carbon hydrogen plants, supported by IRA tax credits and EU innovation grants. In Europe, a joint venture between Thyssenkrupp and Nucera has been steadily growing its order book for green hydrogen used in refining and steelmaking. The Middle East has also emerged as a potential production and consumption base for green hydrogen, as the region seeks to diversify its economy away from oil through projects such as the NEOM Green Hydrogen Project. However, green hydrogen is still thwarted by high capital and operating costs, the need for tailored infrastructure requirements (e.g., existing gas pipelines need to be retrofitted), technical complexities around its efficient storage and transportation (e.g., energy loss when converting to/from ammonia), and uncertainty around newer potential uses in sectors such as utilities or airlines.
Low-carbon aviation fuels are a necessary lever to decarbonizing airlines but will probably only be commercially ready post-2030. Innovation into green plane fuels has focused on two main branches: hydrogen-based fuels and biofuels. The deployment rate of each is likely to depend on their relative costs and competitiveness—while biofuels such as HEFA today win on lower costs, rapid innovation is cutting the costs of hydrogen-based fuels that promise a much higher emissions reduction potential. In March 2023, US company Universal Hydrogen completed its first zero-carbon flight powered by a hydrogen fuel cell. Likewise, ZeroAvia is focusing on hydrogen fuel-cell technology for aviation. It is researching the using of cryo-compressed hydrogen as space-efficient plane fuel and researching modular electrolysis cells that can produce hydrogen on-site in airports to cut the logistical and infrastructural burdens of transporting the fuel. Airbus, for example, has made hydrogen fuel a key part of its decarbonization strategy, but will probably only bring commercial zero-emissions aircrafts to market post-2035. However, even the more mature forms of low-carbon aircraft fuel available today, such as HEFA and gasified feedstock, are either extremely costly (three to five times the cost of traditional fuels) or don’t cut emissions enough, and more innovation will be needed to successfully scale the technology.
The technology supporting plant-based foods is reaching maturity but would require difficult broad-based shifts in diets to be scaled. Animal-based foods produce more carbon and methane while using more land and water than plant-based foods. Meat production is projected to double by 2050, driven by increasing population and incomes. One avenue that can help to decarbonize the sector is by switching to plant-based alternatives, which are already cost-competitive and exist at scale through public companies such as Beyond Meat, which offers lower-carbon alternatives to traditional meat products. Technology aside, the main obstacle to scale is a shift in dietary preferences. In the absence of that, companies are likely to continue to face headwinds at scale, which has been reflected in the significant decline in Beyond Meat’s stock (down more than 80% since IPO). Animal-based products are also major sources of income for populations in emerging markets—as well as some developed economies like New Zealand—which makes it more challenging to pass policy measures curtailing livestock or meat production without exacerbating existing income inequalities.
Novel technologies aimed at reducing livestock emissions, such as enzymes or feed supplements, are still early-stage. Livestock, primarily cows, produce about 15% of global emissions through enteric fermentation that produces methane as a by-product. However, new livestock feeds are being engineered that can improve the digestibility of feed and suppress methane-forming processes in animals’ guts, with methane reductions reported in the range of 25-75%. For example, Syngenta’s genetically modified Enogen corn contains a high amount of amylase, which helps animals digest grain and cuts their methane emissions. Similarly, startups like Mootral and Alltech are developing natural feeds aimed at reducing livestock methane emissions, while others like Symbrosia, Blue Ocean Barns, and CH4 Global are developing algae-based equivalents. That said, these technologies remain earlier-stage and are far from being rolled out at scale.
V. Carbon Capture, Utilization, and Storage: Early Stage but Huge Potential and Critical to Net Zero

After reducing emissions via the other pathways discussed above, residual hard-to-abate emissions in sectors such as cement, steel, oil and gas, and agriculture will need to be absorbed through technologies such as carbon capture, utilization, and storage (CCUS) or similar solutions for methane. The scale-up needed in CCUS for net zero is massive; while exact numbers will depend on how effective other technological solutions are in reducing emissions, the IEA estimates that capacity will need to grow by at least 100 times by 2050 to meet net zero. While point-source CCUS is cheaper and more commercially ready today, the development of direct air capture (DAC) will be needed in the longer term to enable emissions reductions at greater scale, but pilot projects in flight are limited. Concurrently, the industrial uses of captured carbon also need to be developed: currently, the largest demand for captured CO2 comes for enhanced oil recovery, which extends the productive lifespan of oil fields and incentivizes investment in the technology from oil and gas companies. But new uses in other sectors such as building aggregates, chemicals, or synthetic fuels—or a structural shift in demand for low carbon products—will be needed to extend the business case for investment.


Technological development has led to slight cost reductions in point-source carbon capture, but further innovation and policy support will be required to scale projects. Most commercial funding in CCUS to date has gone to point-source carbon capture, which has had moderate success with around 40 commercial facilities currently active. Chevron runs one of the world’s largest carbon capture and storage facilities at its Gorgon LNG plant in Australia, but its capacity is about 4 million tonnes of CO2e annually (less than 1% of Chevron’s reported scope 1, 2, and 3 emissions). Additionally, levelized costs for point-source carbon capture have not decreased substantially over the past decade; however, with the support of policy and new technological innovations, companies in the US and EU are emerging as leaders in deploying point-source CCUS. The IRA in the United States increased the payout of Section 45Q credits on CCUS, which is now sufficient to “neutralize” the incremental costs of adding CCUS across high-emitting industries (although questions remain around permitting). In Europe, the EU Innovation Fund has provided €3.3 billion to companies such as Heidelberg Materials, whose GeZero project aims to produce net-carbon-negative cement by pioneering a new design for a full on-site chain covering the capture, transport, and permanent storage of all its carbon emissions in Germany.
Other methods of carbon sequestration such as direct air capture are currently very early-stage, but have key pilot projects that could unlock larger investment and innovation inflows. Capturing carbon directly from the air, where CO2 concentration is low, tends to be more expensive than point source capture, but has a large emissions reduction potential as carbon can be captured from anywhere rather than a specific plant or facility. Key leaders in this space are Climeworks, Carbon Engineering, and Carbfix. The first commercial-scale DAC plant by Carbon Engineering (acquired by Occidental and backed by BlackRock) in Texas could mark a turning point for its scalability, and aims to bring down costs from $600 to between $94 and $232 per tonne of CO2 sequestered. Frontier—a public benefit company co-founded by Stripe, Alphabet, Shopify, Meta, and McKinsey—is another company aiming to “jump start” the carbon capture market by pioneering a new model of pooled funding.
Apart from direct air capture, pilot projects are also underway on other types of carbon capture technologies such as soil-based sequestration, bioenergy with carbon capture, and enhanced rock weathering. These are potentially cheaper than direct air capture but risk creating adverse natural capital impacts on biodiversity, food security, or ocean chemistry. For example, take-up of soil-based sequestration technologies by farmers has been slow because of the risk it poses to near-term crop yields, despite them being able to convert the sequestered carbon into voluntary credits.
Methane (and other greenhouse gases) capture and leakage minimization are mature technologies and key in industries such as oil and gas. Methane makes up about 15% of global emissions and has a higher short-term global warming potential than carbon dioxide, due to its greater potency (about 80x) as a greenhouse gas. Major oil companies (e.g., Shell, TotalEnergies, BP, Saudi Aramco, Petrobras) have signed onto a pledge at COP28 to slash methane pollution. Vapor recovery units are already used to capture methane from storage tanks that would otherwise be “vented” or released into the atmosphere and can reach payback in as little as two months from increased natural gas savings. Other methods to detect and repair methane leaks (e.g., infrared cameras) are also commonly used, driven by government and international policy (such as the World Bank’s Global Flaring and Methane Reduction Partnership or recent EPA regulations) and increased scrutiny of oil and gas companies via satellite monitoring of methane leaks.
Methane is also a large by-product of agriculture and livestock—which account for roughly a third of anthropogenic methane emissions—but technologies here are less mature. For example, pilot projects are underway that use methane digesters, which are sealed tanks that capture the gases created when manure, crop residues, or other organic waste is broken down and convert it into biogas instead of releasing it into the atmosphere. California—as the United States’ largest dairy-producing state—is an emerging innovation hub, spending $350 million to produce 120 digesters since 2015 (with plans for another 230 by 2030) in partnership with startups such as Maas Energy Works and CalBio. The resultant biogas is used primarily for electricity and heat, but levelized costs remain higher than other electricity sources, which limits the scalability of new projects.
This research paper is prepared by and is the property of Bridgewater Associates, LP and is circulated for informational and educational purposes only. There is no consideration given to the specific investment needs, objectives, or tolerances of any of the recipients. Additionally, Bridgewater's actual investment positions may, and often will, vary from its conclusions discussed herein based on any number of factors, such as client investment restrictions, portfolio rebalancing and transactions costs, among others. Recipients should consult their own advisors, including tax advisors, before making any investment decision. This material is for informational and educational purposes only and is not an offer to sell or the solicitation of an offer to buy the securities or other instruments mentioned. Any such offering will be made pursuant to a definitive offering memorandum. This material does not constitute a personal recommendation or take into account the particular investment objectives, financial situations, or needs of individual investors which are necessary considerations before making any investment decision. Investors should consider whether any advice or recommendation in this research is suitable for their particular circumstances and, where appropriate, seek professional advice, including legal, tax, accounting, investment, or other advice.
The information provided herein is not intended to provide a sufficient basis on which to make an investment decision and investment decisions should not be based on simulated, hypothetical, or illustrative information that have inherent limitations. Unlike an actual performance record simulated or hypothetical results do not represent actual trading or the actual costs of management and may have under or overcompensated for the impact of certain market risk factors. Bridgewater makes no representation that any account will or is likely to achieve returns similar to those shown. The price and value of the investments referred to in this research and the income therefrom may fluctuate. Every investment involves risk and in volatile or uncertain market conditions, significant variations in the value or return on that investment may occur. Investments in hedge funds are complex, speculative and carry a high degree of risk, including the risk of a complete loss of an investor’s entire investment. Past performance is not a guide to future performance, future returns are not guaranteed, and a complete loss of original capital may occur. Certain transactions, including those involving leverage, futures, options, and other derivatives, give rise to substantial risk and are not suitable for all investors. Fluctuations in exchange rates could have material adverse effects on the value or price of, or income derived from, certain investments.
Bridgewater research utilizes data and information from public, private, and internal sources, including data from actual Bridgewater trades. Sources include BCA, Bloomberg Finance L.P., Bond Radar, Candeal, CBRE, Inc., CEIC Data Company Ltd., China Bull Research, Clarus Financial Technology, CLS Processing Solutions, Conference Board of Canada, Consensus Economics Inc., DataYes Inc, Dealogic, DTCC Data Repository, Ecoanalitica, Empirical Research Partners, Entis (Axioma Qontigo Simcorp), EPFR Global, Eurasia Group, Evercore ISI, FactSet Research Systems, Fastmarkets Global Limited, the Financial Times Limited, FINRA, GaveKal Research Ltd., Global Financial Data, GlobalSource Partners, Harvard Business Review, Haver Analytics, Inc., Institutional Shareholder Services (ISS), the Investment Funds Institute of Canada, ICE Derived Data (UK), Investment Company Institute, International Institute of Finance, JP Morgan, JSTA Advisors, M Science LLC, MarketAxess, Medley Global Advisors (Energy Aspects Corp), Metals Focus Ltd, Moody’s ESG Solutions, MSCI, Inc., National Bureau of Economic Research, Neudata, Organisation for Economic Cooperation and Development, Pensions & Investments Research Center, Refinitiv, Rhodium Group, RP Data, Rubinson Research, Rystad Energy, S&P Global Market Intelligence, Scientific Infra/EDHEC, Sentix GmbH, Shanghai Metals Market, Shanghai Wind Information, Smart Insider Ltd., Sustainalytics, Swaps Monitor, Tradeweb, United Nations, US Department of Commerce, Verisk Maplecroft, Visible Alpha, Wells Bay, Wind Financial Information LLC, Wood Mackenzie Limited, World Bureau of Metal Statistics, World Economic Forum, and YieldBook. While we consider information from external sources to be reliable, we do not assume responsibility for its accuracy.
This information is not directed at or intended for distribution to or use by any person or entity located in any jurisdiction where such distribution, publication, availability, or use would be contrary to applicable law or regulation, or which would subject Bridgewater to any registration or licensing requirements within such jurisdiction. No part of this material may be (i) copied, photocopied, or duplicated in any form by any means or (ii) redistributed without the prior written consent of Bridgewater® Associates, LP.
The views expressed herein are solely those of Bridgewater as of the date of this report and are subject to change without notice. Bridgewater may have a significant financial interest in one or more of the positions and/or securities or derivatives discussed. Those responsible for preparing this report receive compensation based upon various factors, including, among other things, the quality of their work and firm revenues.